The Past, Present, and Future of Faraway Worlds - The Ringer
Published by Reblogs - Credits in Posts,
The Past, Present, and Future of Faraway Worlds - The Ringer
Filed under:
The Past, Present, and Future of Faraway Worlds
Thirty years ago this month, astronomers first confirmed the existence of planets beyond our solar system. The anniversary comes as NASA’s ultra-powerful James Webb Space Telescope gets ready to begin its work, raising the question: What kinds of extrasolar discoveries await?
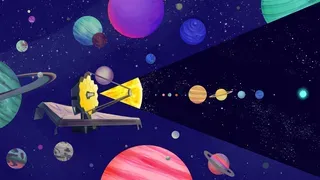
Share this story
There is a single general space, a single vast immensity which we may freely call Void; in it are innumerable globes like this one on which we live and grow. This space we declare to be infinite, since neither reason, convenience, possibility, sense-perception nor nature assign to it a limit. In it are an infinity of worlds of the same kind as our own. —Italian cosmological theorist Giordano Bruno, 1584 (16 years before he was burned at the stake)
The Milky Way holds wonders that would make the strangest sights in science fiction seem uninspired. Our planet and solar system may be special in some ways—we’re here, for one—but in other respects, our sliver of space can’t compare to the galaxy’s extremes. "As creative as your imagination can be, what we’ve learned from space exploration … is that nature is far more creative," planetary scientist Heidi B. Hammel says.
About 1,800 light-years from Earth, there’s a super-Jupiter skimming so close to the surface of its frightful host star that the planet’s year lasts less than a day, and the gravitational tug it experiences stretches it into an egg shape. Only 40 light-years away—much nearer to our neighborhood, yet still almost unimaginably remote—is a planet whose hot side attains temperatures close to 3,000 degrees and whose cold side sinks hundreds of degrees below zero. Equally distant, in a different direction, is a super-Earth that may (or may not) be made of diamond. There are, we know now, planets with three local stars in their skies—and, it seems, "circumtriple" planets that actually orbit three stars. There are "super-puff" planets no denser than cotton candy, and planets that rain molten iron and glass, and planets that are bigger than the stars they circle (not to mention free-floating rogue planets that don’t circle stars at all).
Perhaps the only thing more mind-melting than the mélange of thousands of extrasolar planets (or exoplanets) whose existence has been documented is that it’s still possible to call up the people who found the first ones. Some Americans not old enough to run for president in 2024 were born into a world where the only planets our species had discovered were the ones around the sun. The first confirmed detection of a planet that’s not next door (astronomically speaking) was not announced until 30 years ago this month. And as humanity marks that milestone, a newly launched telescope—and recently published plans for even more powerful future scopes—are poised to make the next three decades of exoplanet-hunting far more fruitful than the last. Our knowledge of other worlds than these has expanded exponentially, from zero to almost 5,000, with many more tentative detections waiting to be corroborated. (Five new exoplanets dropped just this week.) But the Age of Exploration in an extrasolar sense has only just begun.
This week, NASA’s James Webb Space Telescope arrived at L2, the second Sun-Earth Lagrange point, a region in space nearly 1 million miles opposite the sun from Earth where celestial mechanics make it possible for objects to stay in stable orbits. The telescope, which launched on Christmas morning, spent the trip unfolding its sunshield and its 21-foot (6.5-meter) gold-plated primary mirror in a painstaking, anxiety-inducing sequence of hundreds of successfully executed steps. The $10 billion instrument, which took decades to develop, is approximately 100 times more sensitive to infrared light than the Hubble Space Telescope, giving it—as an oft-cited spec says—the capacity to pick up the heat of a bumblebee at the distance of the moon. It would have been a big waste to spend $10 billion on an ultra-long-range bumblebee detector, given that there aren’t any bumblebees on the moon. (And if there were, they wouldn’t stay warm for long.) So Webb will instead use that power to study some of the oldest and most distant structures in the universe, rewinding the record closer to the Big Bang by probing the formation of the first stars and galaxies. It’s also expected to spend about a quarter of its roughly 20-year operational lifetime peeking at exoplanets.
Home, home on Lagrange! We successfully completed our burn to start #NASAWebb on its orbit of the 2nd Lagrange point (L2), about a million miles (1.5 million km) from Earth. It will orbit the Sun, in line with Earth, as it orbits L2. https://t.co/bsIU3vccAj #UnfoldTheUniverse pic.twitter.com/WDhuANEP5h
— NASA Webb Telescope (@NASAWebb) January 24, 2022
The Webb went through a multitude of delays and cost overruns because it’s one of the most precise and sophisticated machines ever designed. The same can’t be said about Alex Wolszczan’s first telescope. Wolszczan, a professor of astronomy at Penn State’s Eberly College of Science, grew up in postwar Poland, where he learned a love of the heavens from his father, who taught him the legends that gave the constellations their names. As a young boy, he constructed a small telescope out of glasses usually used in eyeglasses and microscope eyepieces. Gazing upward from his attic, he saw the rings of Saturn, as well as Jupiter and its Galilean moons—the first objects ever found to orbit a planet other than Earth. Galileo beat him to those sights by more than 300 years, but the spectacle inspired him to pursue astronomy in hopes of seeing more. "It was really a desire to discover things up there," he says.
About 40 years later, he’d discover something that was way beyond the abilities of Galileo, Johannes Kepler, or Nicolaus Copernicus, whose name adorns the school where Wolszczan studied astronomy: the first extrasolar planets. "The story of planet detection goes back quite a bit," Wolszczan says. The first evidence of an exoplanet was actually captured in 1917 but wasn’t recognized as such for a century. Some false alarms followed in the 1940s and ’60s. In the mid-1980s, using instruments such as the space-based Infrared Astronomy Satellite—an early ancestor of Webb—astronomers discovered evidence of protoplanetary disks, the haloes of dense gas and dust surrounding stars that can compact into planets. In 1988, a team of astronomers announced that they’d discovered an exoplanet, only to retract the claim later. (Fifteen years after the initial announcement, follow-up observations showed that the planet actually was there.) And in 1989, an astronomer reported a possible exoplanet that wasn’t confirmed until 2012.
But the first astronomer to announce a no-fooling exoplanet that existed beyond doubt was Wolszczan, in January 1992. "There was a lot of randomness and good luck and accident involved in all this, because technologically everything was ready to go," he says. "That sort of discovery could have been made 10 years before or so, if someone really thought about doing what I decided to do."
Wolszczan’s discovery relied on the properties of celestial phenomena called millisecond pulsars, the first of which was discovered in 1982. Millisecond pulsars are small but incredibly dense neutron stars—the collapsed cores of supergiant stars, left over after they explode in supernovas—that spin so fast that they complete a full rotation in less than about 10 milliseconds. Their gravity and magnetic fields are so strong that nearby material slams into them at scary speeds, producing focused beams of energy that they emit like lighthouses. As the neutron stars spin, those beams look like pulses as seen from Earth. The pulses are so astonishingly regular that any minute deviation can indicate the presence of a foreign object whose gravity is exerting itself on the star.
In January 1990, the world’s largest and most sensitive radio telescope, located at the Arecibo Observatory in Puerto Rico—known to non-astronomers as the GoldenEye dish—underwent repairs. Unable to change its orientation, it could observe only what it happened to be pointed toward by the Earth’s rotation. That diminished mobility ruled out certain research and cut down on demand for telescope time. Wolszczan took advantage of the extra time to search for millisecond pulsars, and in February, he found two, one of which was a binary that initially seemed more interesting than the other because of its potential to test Einstein’s theory of relativity. But the solitary pulsar proved puzzling. When Wolszczan went back to catalog it, he found that its timing varied in an abnormal way. "I just couldn’t get the standard timing model for a pulsar to work," Wolszczan says. "Then over time, it turned out that the reason was that there were two Earth-mass, or super-Earth-mass [exoplanets] orbiting it."
Pulsars weren’t thought to be capable of supporting planets, because the stellar explosions that formed them would have destroyed any nearby objects. (In fact, reconstituted planets can coalesce around pulsars, potentially formed from matter that fails to escape the vicinity of the supernova, or from a merger of two white dwarfs that produces a pulsar with a surrounding disk.) But the math, augmented by independent observations made by colleague Dale Frail at the Very Large Array—known to non-astronomers as the dishes from Contact—was inarguable. "I made a joke to Alex saying, ‘And don’t go finding any planets, smiley face,’" Frail recalls. "And he emailed me back saying, ‘Two planets, period this, period that.’ And I thought he was joking. … It was just mind-blowing."
Our first VLA image of PSR B1257+12 from July 1991. The VLA position, ~1000x better than Arecibo's initial one, refined Arecibo’s timing solution and helped lead to the discovery of the first two pulsar planets. Published in Nature 30 years ago today. #exoplanets #NRAO #Arecibo pic.twitter.com/he8Q5SGOSo
— Dale A. Frail (@DaleFrail) January 9, 2022
Galileo could see his discoveries, and the young Wolszczan had been hooked by the spheres he glimpsed through his makeshift eyepiece. The pulsar he’d found, PSR B1257+12, was 2,300 light-years away, its planets too dim and distant to be visible. Yet he felt the same thrill of discovery when he realized that slightly elliptical orbits would explain some small inconsistencies in a model he’d made that had fitted the two planets with circular orbits. "Everything fell in place perfectly," he says, adding, "That was like this eureka moment for me. … It had to be planets. That was it."
Wolszczan and Frail published a paper in Nature and, soon after, headed for Atlanta to present the findings at the annual meeting of the American Astronomical Society. All along, they thought they’d been beaten to the pulsar-planet punch by the University of Manchester’s Andrew Lyne, who had announced a similar find the previous summer. "It’s a very human thing to be disappointed in [that] situation," Wolszczan says. "But at the same time, I was so fascinated by the situation that it didn’t really hit me that hard. It was like, ‘Well, so be it. I’ll be the second one, not the first.’" But Lyne shocked the crowd at the AAS meeting by retracting his results. What had appeared to be a planet was—ironically, given Wolszczan’s epiphany—an artifact of a failure to fully account for the Earth’s slightly elliptical orbit. Lyne’s mistake meant that Wolszczan and Frail were first after all, which earned Wolszczan a place in a set of Polish stamps that also featured Copernicus. Just as Copernicus had shown that the Earth revolved around the sun, puncturing some of our species’ geocentric exceptionalism, Wolszczan had conclusively demonstrated that our solar system wasn’t alone in harboring planets.
Wolszczan continued to refine his measurements of the historic system he’d found, and in 2005, he announced the discovery of another, significantly smaller planet orbiting the same star, PSR B1257+12 A, which is the least-massive exoplanet (or planet, period) discovered to date. That comparatively tiny body is only about .02 times Earth’s mass, while PSR B1257+12 B and PSR B1257+12 C check in at 4.3 and 3.9 Earth masses, respectively. Even though Wolszczan can’t really look at his discoveries, he does have an image in his mind’s eye of the pulsar (which is named Lich) and the three planets (which are named Draugr, Poltergeist, and Phobetor). He pictures "gleaming surfaces in this eerie, strange light," as particles from the pulsar produce glazed landscapes lit by permanent auroras bright enough to read by.
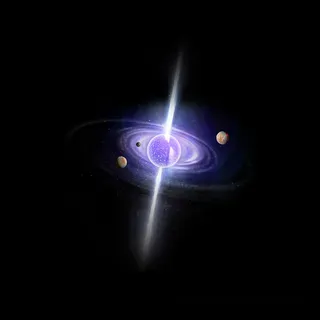
The discovery of the planets orbiting Lich was a crucial link between the speculative period of unproven planet-hunting and the subsequent ascendancy of exoplanetology. "The early days of extrasolar planet stuff was—I wouldn’t say the word fringe, but was difficult, out-there science," says Frail, who adds, "It’s very hard for someone now, with 5,000 planets out there, to think about … how nascent the field was and how the people legitimately did not know whether there were planets around other systems." Contrast that to the current state of planetary studies, as described by Hammel, an experienced observer of the outer solar system who’s now serving as an interdisciplinary scientist on James Webb. "I knew things had changed a few years ago when somebody said, ‘What field do you work in?’" she says. "And I said, ‘Planetary science.’ And they said, ‘Oh, what’s your favorite exoplanet?’ And I’m like, ‘Oh, I actually mean planetary science, like planets in our solar system.’ And it hadn’t even occurred to them that there was planetary science in our solar system, because it was all exoplanets."
In some respects, the characteristics of the first known extrasolar planetary system presaged discoveries to come: It featured resonant, tightly packed planets and two super-Earths, which we’ve subsequently learned are common attributes of extrasolar systems. On the other hand, millisecond pulsars are rare, as are pulsar planets (not many more of which have been found). In a sense, the system’s weirdness was encouraging. "I remember some really well-established planet people saying, ‘Look, we can find them around pulsars,’" Frail says. "‘They’ve got to be around pretty much any other star.’" Others, Wolszczan says, considered the system an irrelevant outlier. "People who were engaged in various programs to search for planets around normal stars basically dismissed it. They said, ‘Oh, this is an interesting curiosity, but this is not what we are really looking for.’"
Not that anyone knew for sure what they’d find. In the beginning, it was widely believed that the most common (and most easily observable) type of exoplanet would be massive gas giants that, like Jupiter, would orbit at a great remove from their stars. It’s true that larger exoplanets are easier to detect: Of those with known radii, only 13 percent are less than or equal to 1.25 times Earth’s size, and of those with known masses, only 5 percent are less than or equal to 3 times Earth’s mass. (By the most generous estimate, only 59 known exoplanets are thought to be potentially habitable.) But to most astronomers’ surprise, many of the early exoplanets turned out to be "hot Jupiters" that gradually migrated inward to orbit quite close to their stars. The first exoplanet discovered around a main-sequence star—the kind that’s still sustainably fusing hydrogen into helium, as stars do throughout most of their lifespans—was a hot Jupiter called 51 Pegasi b, which orbits a sun-like star about 50 light-years away. That 1995 find was awarded a Nobel Prize in Physics in 2019. (Wolszczan is still waiting for a call from the committee.)
Our solar system doesn’t have a hot Jupiter (though Jupiter itself could have become one), which illuminates the dangers of extrapolating from a sample of one. "What I’ve found most fascinating about the field of exoplanets is how it has really turned our understanding of planetary systems upside down in terms of how planetary systems form," Hammel says. "We had a story based on our solar system, but the thousands of planets that we’re finding now are showing us that our planetary system is but one kind of planetary system, and not even a typical kind of planetary system."
Another way in which Wolszczan’s discovery lies off the main sequence of exoplanet research is that fewer than 10 total exoplanets have been found via variations in pulsar timing. More than three-quarters of confirmed exoplanets have been discovered via the "transit method," in which an instrument such as NASA’s Kepler space telescope (which was responsible for more than half of all exoplanet discoveries to date) or Transiting Exoplanet Survey Satellite (TESS) detects a tiny, telltale dimming in the luminosity of a star as a planet passes in front of it as viewed from Earth, allowing us to learn about the planet’s size and atmospheric composition.
Only a small fraction of exoplanets transit their star from our planet’s perspective, but because there are so many exoplanets out there—approximately one or two, on average, for each of the 200 billion to 400 billion stars in our galaxy alone—even an inefficient dragnet can catch a lot of candidates. About a tenth of those stars are similar to the sun, and as many as half of those could have habitable planets. Elisa Quintana, who in 2014 led the team that discovered the first definitive Earth-sized exoplanet in the habitable zone of another star (and now leads the Exoplanet Group at NASA Goddard), says Kepler’s "goal was to see if Earths were out there and if they were common, so it was a statistical survey. And it turned out that Earths were everywhere."
Almost 20 percent of confirmed exoplanets—including 51 Pegasi b—have been detected by the radial velocity method, the dominant method prior to Kepler’s launch. This approach relies on measuring minute shifts in the speed with which a star moves toward or away from Earth as a result of the gravitational pull of a planet. Many more methods have been used to add to the exoplanet library, including gravitational microlensing, in which the gravitational fields of a star and its planet(s) serendipitously magnify the light of an even more distant star in a way that allows the planet’s presence to be discerned. Direct imaging of exoplanets is also becoming increasingly viable, although their distance and dimness relative to their stars makes observing them directly difficult.
Some tools and tactics (like the transit method) used to study bodies in our solar system have been borrowed and repurposed for exoplanet science, and some discoveries have been duplicated. Hammel remembers early exoplanet sessions at AAS meetings when astronomers would breathlessly report that, for instance, an exoplanet’s atmosphere was hotter higher up. "And those of us who are planetary scientists are like, ‘Well, you have discovered the stratosphere. Congratulations. This is not a new and surprising thing. All of the giant planets have warm stratospheres.’" But thanks to ever-advancing computers and telescopes, exoplanet science soon set a faster pace than the early study of our solar system. "The progress has been so rapid in comparison because our tools are so much more sophisticated now," Hammel says.
Like the number of transistors in a circuit, the number of known exoplanets has doubled roughly every two years. Astronomers are now working on confirming the first exoplanet outside our galaxy—an exoexoplanet, if you will—and the first exomoon. With our telescopic powers combined, the count and variety of detections will keep climbing, and several new space- or ground-based instruments devoted to detecting or characterizing exoplanets are scheduled to come online later this decade. (Granted, we’ve lost some tools, too: The venerable Arecibo radio telescope collapsed in December 2020, about which Wolszczan says, "It was hard not to feel somewhat emotional.")
Webb, which has fully deployed its mirror and telescope-sized sunshield, is currently cooling down to a frigid temperature suitable for infrared observing and will spend the coming months aligning and calibrating its instruments in preparation for first light. The telescope isn’t expected to discover a huge number of new exoplanets, though it will discover some. Its main contribution to the field will be using its spectrograph to examine the light passing through exoplanets’ atmospheres for markers of interesting elements, such as water, oxygen, carbon dioxide, or methane, that could indicate life or possible by-products of life—the thrilling last step in humanity’s incremental, millennia-long demotion from self-conceived masters of the universe. "Although you can never be certain of anything, the biosignatures we’re choosing to look for are sort of the most unambiguous ones," says Stanford professor of physics and exoplanet imaging expert Bruce Macintosh.
#NASAWebb is safely in space with its solar array drawing power from the Sun! Its reaction wheels will keep the spacecraft pointed in the right direction so that its sunshield can protect the telescope from radiation and heat: https://t.co/NZJ7sSJ8fX#UnfoldTheUniverse pic.twitter.com/s4nfqvKJZD
— NASA Webb Telescope (@NASAWebb) December 25, 2021
Webb will, for instance, be able to study TRAPPIST-1, a red dwarf star 39 light-years away that boasts an impressive seven terrestrial planets, three or four of which lie within the star’s habitable zone, the region where temperatures support liquid water on the surface (which isn’t necessarily a prerequisite for life, let alone a guarantee of it). "Webb is really going to start providing a treasure trove of data for this system, but we’re just not going to get to the bottom of the treasure chest yet," says Knicole Colón, JWST’s deputy project scientist for exoplanet science. "We’re going to start digging coins off the top."
The full Scrooge McDuck–style deep dive into potentially habitable (or inhabited) planets lies decades down the road. "In Webb’s lifetime, I think we’ll be able to start to have good evidence for some of these things or rule out evidence," Colón continues. "But that’s where these future missions come in, where we need additional data at other wavelengths as well to complement the Webb data and say, ‘OK, now we have independent verification.’"
Last November, the National Academies of Sciences, Engineering, and Medicine released the pandemic-delayed Decadal Survey on Astronomy and Astrophysics 2020, or Astro2020, a 600-plus-page summation of American astronomers’ recommendations to Congress, NASA, and other government entities that help control the course of space exploration. This latest incarnation of the Decadal doesn’t confine itself to the next 10 years. Instead, it charts out a possible path through the next 20 to 30 years. "It sets out a longer-term road map," says Macintosh, who served on the Survey’s steering committee. "In some ways, what it might be is more upfront about that than previous Decadals. … This is just recognizing that these things take 20 years, so that we have to be explicit about laying out plans."
Previous Decadals spurred the development of Hubble, JWST, and the forthcoming Nancy Grace Roman Space Telescope. The new Decadal calls for a space-based, multi-wavelength scope more than twice as large as Hubble, as well as two ground-based telescopes that would be three times bigger and 100 times more sensitive than any in operation now. The pressure remains on JWST to do exciting science that will generate enthusiasm for future 11-figure expenditures. "JWST’s continuing successes will help a lot with everything from congressional politics down to individual astronomers too, [who] will start to remember that big ambitious flagships really can do important, transformative things," Macintosh says.
Sign up for the The Ringer Newsletter
Technology has already revolutionized planetary science in the span of a still-active career. When Hammel entered the field in the late ’80s, she says, "We hadn’t even done a first reconnaissance of our solar system and the planets. Uranus and Neptune were just a little bit more than a dot in the sky, kind of like what we see with exoplanets now." Hammel was part of the team that imaged Neptune—then the most far-flung planet known, excluding the subsequently de-planeted Pluto—from NASA probe Voyager 2, which completed the first (and thus far only) flyby of the sun’s eighth planet in 1989. "I had spent years studying these blobs, little fuzzy blobs, trying to imagine in my mind’s eye, what could this possibly be?" she says. "And then Voyager was revealing it all to us."
A few decades hence, the same scales may fall from exoplanetologists’ eyes. "Even from a fuzzy dot, we can get a lot of information and extract what the atmosphere of that planet is made of," Colón says. But exoplanets, unlike Bigfoot, won’t be fuzzy forever. The Decadal’s dream telescopes, or more exotic solutions like a lunar telescope, a solar gravitational lens, or a reboot of the Space Interferometry Mission, could let us see the glint of oceans or the hue of flora. Colón continues, "I’m confident we could get to the point where it’s not necessarily just a blob, but where you could see evidence, at the very least, of dark blobs versus lighter blobs, let’s say land versus water." Or, she adds, "Maybe you can make out that there’s something orbiting the planet, and it’s definitely not a moon." While we’re shooting for the not-a-moon, we might as well go full sci-fi: "People talk about James Webb looking for oxygen in somebody’s atmosphere, but James Webb could also find super civilizations," says SETI Institute astronomer Seth Shostak.
If the next generation of telescopes will so potently surpass the current cutting edge, one might wonder why astronomers don’t just twiddle their thumbs until technology improves. After all, Macintosh says, "Everything … in my PhD advisor’s PhD thesis, which was like six years of his life with state-of-the-art equipment, I could do in five minutes with a 24-inch telescope and a [smartphone]." But as Macintosh notes, "That doesn’t mean it’s bad that he did that. People would like to know the answers and not wait. You advance science with the technologies you have. Building one generation of equipment informs building the next generation and also scientifically informs the kind of questions that you ask."
The cost and complexity of studying exoplanets might leave the more results-oriented among us with one question: Why are we doing this? The closest confirmed exoplanet, Proxima b, is—like other possible exoplanets that may orbit the stars closest to the sun—more than four light-years away. (To put that vast span into perspective, Neptune is about four light-hours away.) Why devote so much time, money, and effort to peering at places we can’t visit or settle, can’t harvest for resources, can’t even theoretically communicate with except potentially on timescales of decades or centuries? What’s in it for us?
One reason to reconnoiter is that we are, in all likelihood, extraordinarily lucky to be alive, which we shouldn’t take for granted. "By studying the full range of possible planets, particularly planets around stars like our own, we are able to build up a more solid understanding of how habitable planets have come to be," Hammel says. "If we find another habitable planet out there, that will help us understand the story of our solar system and how our planet became habitable. And it might give us clues as to the end game for our solar system." As author and astronomer Phil Plait put it this week in discussing three exoplanets that may be doomed to be swallowed by their star, "Learning about their fate may very well reveal something about ours."
That may be true in an abstract sense, but your fate and mine—and even that of our species—will be settled billions of years before the expanding sun incinerates the Earth. We don’t need to know, and yet something inside us demands to. Thus, the exoplanet search speaks to more fundamental matters. "Do you answer scientific questions only because they have direct, tangible, practical, financial relevance to human beings or not?" Macintosh asks. "I believe, extremely strongly, that exploring fundamental scientific questions like, ‘Is life in the galaxy rare? Is the process that led to life on the Earth common or unique? How did life arise on the Earth? How’s it going to evolve in the future?’—I think answering fundamental questions like that is a grand human value and has been for thousands and thousands of years."
Just as George Mallory supposedly said he wanted to climb Mount Everest because it was there, scientists search for exoplanets because they’re there (or might be there). The fact that we’re trying to find them even though they’ll be unreachable for the foreseeable future makes the pursuit one of the purest possible expressions of our hardwired desire to understand the workings of our world (and other worlds). And just as Wolszczan surprised the scientific community by finding pulsar planets, those who work with Webb and its successors will probably break ground that no one knew was there.
"What the history of astronomy and science in general teaches us is that nearly every time you come up with a new instrument or new experiment that really outperforms what has been done in the past, then perhaps it is not most interesting what you predict is going to be discovered, but what you have no idea [will be discovered]," Wolszczan says. "This is really what I’m most excited about: Just expect the unexpected." ■
Spotify’s Joe Rogan Problem, Facebook’s TikTok Crisis, and Crypto’s Anonymity Conundrum
Tags: Space